Many sources of sustainable energy such as solar cells or wind turbines produce fluctuating levels of electricity, motivating the search for means to intermittently store the energy. The (photo)electrochemical generation of hydrogen from water represents an eco-friendly and sustainable means of energy generation and storage. With a focus on developing specific nanostructures that can beneficially orchestrate the flow of charge carriers and molecules, the group is investigating different design strategies for the (photo)electrodes.
It turns out that the conversion of solar energy in (photo)electrochemical processes such as water splitting depend critically on the structure, composition and interfaces of the active materials. For example, the light-driven water-splitting on semiconducting metal oxides is an attractive method for generating hydrogen fuel in a sustainable way. The efficiency of this process is, however, far below theoretical predictions due to different loss mechanisms in photoelectrode materials, including a high overpotential for the overall water splitting reaction.
Exploring semiconductors featuring structural and chemical tunability combined with high solar-to-chemical energy conversion efficiencies, provided by organic semiconducting polymers is expected to advance the (photo)electrochemical water reduction to hydrogen. More specifically, the rise of covalent organic frameworks (COFs), have opened new possibilities to improve the current performance of (photo)electrocatalysts. Our group has developed various deposition techniques such as the in-situ growth [1], vapor-assisted conversion (VAC) [2] and electrophoretic deposition (EPD) [3] to generate COF based photoelectrodes which were successfully applied, pristine or loaded with Pt catalyst, for hydrogen generation. In addition, the group made first successful steps towards the growth of the Pt nanoclusters in the pores of the COF and their positioning at the COF’s active sites by applying a photodeposition method.
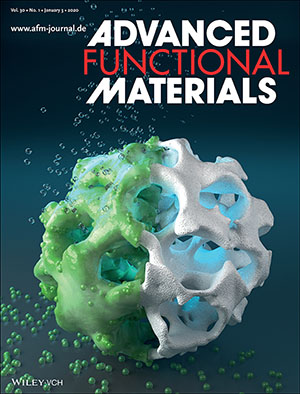
Turning our attention to Proton exchange membrane (PEM) electrolysis, a method of direct water electrolysis into molecular hydrogen and oxygen, can tolerate both fast load changes as well as operation at partial load, which makes it an ideal complement for fluctuating sources of renewable electricity. However, its large-scale deployment is impeded by the requirement for a high loading of the oxygen-evolving electrode with rare and expensive iridium oxide catalyst for sufficient activity and conductivity. In principle, the required amount of IrO2 can be reduced by supporting it on a nanostructured (and ideally conducting) oxide such that very low loadings of the rare metal could become accessible.
We are developing strategies for the generation of PEM oxygen evolution catalysts comprising highly-structured conductive oxide supports and ultra-low IrO2 loading [4, 5]. Different designs of the oxide supports have been developed in the group, for example scalable spray-dried templated macroporous oxide morphologies and electrospinning high surface area oxides. The group has established various deposition techniques (e.g., wet chemical process [4], solvothermal treatment [5], incipient wetness impregnation, and Atomic Layer Deposition [6]) to coat the support with extremely thin layers of the rare iridium oxide catalyst.
[1] T. Sick et al. J. Am. Chem. Soc. 2018, 140, 2085.
[2] E. Virmani. et al. J. Am. Chem. Soc. 2018, 140, 4812.
[3] J. Rotter. et al. Chem. Mater. 2019, 31, 10008.
[4] Böhm et al. Appl. Mater. Today. 2021, 24, 101134.
[5] Böhm et al. Adv. Funct. Mater.2020, 30, 1906670.
[6] Hufnagel et al. Nanoscale, 2019,11, 14285.